2 The Principles of Electricity
Learning Objectives
Describe the basic principles of electricity.
Before you can understand how electrical devices and systems operate, you must have an understanding of the basic principles that govern the behaviour of electricity. In this learning outcome you will gain a foundation for further learning in this course.
It is impossible to understand electricity or the electrical properties of materials without some basic understanding of the structure of matter.
Matter
Matter is anything that occupies space or has mass. It can exist in different forms because of differences in the motions and forces of the elementary particles (molecules, atoms or ions) of which it is composed. Matter can exist in four different states: solid, liquid, gas, and plasma.
Note: The states of matter are also known as phases of matter or states of aggregation.
The molecules of a solid are limited to vibration about a fixed position, so all solids have a definite shape and volume. When a solid is heated its molecules begin to vibrate more rapidly and eventually break out of their fixed positions. When this happens the material has been heated to its melting point, and it becomes a liquid.
The molecules of a liquid are free to move within the liquid but cannot escape from it because of the intermolecular forces of cohesion and adhesion. All liquids have a definite volume but can have any shape. They take on the shape of their container. When a liquid is heated, some molecules gain enough energy to break free into the surrounding space. When this happens the liquid has been heated to the boiling point and evaporates into a gas.
The molecules of a gas are free to move in every possible way, so a gas has no definite shape or volume. When a gas is heated, its molecules may move so violently that they break into their component atoms and some of these atoms lose individual electrons. When this happens, the gas has become plasma, a very hot mixture of electrons and positive ions with different properties than the more common states of matter.
Elements and Compounds
The different types of matter are classified into separate categories called substances, each with its own unique physical properties. A substance may be an element or a compound.
An element contains only one type of atom. There are 92 naturally occurring atoms/elements, known at this time, and at least another 14 man-made elements. All matter in the universe is made from these elements, individually, or more often, in various combinations. Examples of elements include oxygen, hydrogen, carbon, copper, and silver.
A compound contains atoms of more than one element. The atoms have combined to form molecules, and the resulting compound usually has very different properties than the original elements. Water, for example, is a liquid (at room temperature), but is composed of hydrogen and oxygen, which are both gases. Another example is sugar which consists of the elements carbon, hydrogen, and oxygen.
Atoms and Molecules
An atom is the smallest unit into which an element can be broken and still possess the properties of that element. Atoms are assigned letter symbols such as H (hydrogen), O (oxygen), and C (carbon).
A molecule is the smallest unit into which a substance can be broken and still possess the properties of that substance. Molecules are given chemical descriptions that indicate the number and type of atom they contain. For example, water is called H2O because each water molecule contains 2 hydrogen and 1 oxygen atoms. Household sugar is C12H22O11 and contains 12 carbon, 22 hydrogen, and 11 oxygen atoms. Removing or adding even one atom, of any type, would change the nature of the molecule.
An individual atom can never be anything but an element, but molecules are found in both elements and compounds. In an element, there is only one type of atom, but they often combine with each other to form molecules. In a compound the molecules contain atoms from at least two different elements.
Protons and Electrons
Note: The following description is based on a simplified model of the atom. It is crude and incomplete, but adequate for our purposes.
An atom is composed of a dense central nucleus inside a thin cloud of orbiting electrons. The nucleus contains one or more protons, which carry a positive electrical charge, and may also contain one or more neutrons, which carry no electrical charge. The electrons carry a negative charge of the same magnitude as the positive charge on the proton.
All electrical charges are surrounded by an electric field that behaves something like a magnetic field. When two bodies have opposite electrical charges, their fields combine and try to pull them together. When the charges are the same, the interaction of the two electric fields tries to force them apart. As a result, like electrical charges repel, and unlike electrical charges attract.
A powerful nuclear force binds the nucleus together, overcoming the mutual repelling force of the positively charged protons that would otherwise blow it apart. The total positive charge of the nucleus pulls on the negatively charged electrons, holding them in their orbits. Because there are equal numbers of protons and electrons, the net charge on the atom is zero.
Protons are heavy, and trapped within the nucleus. Electrons are extremely light, and travel at very high speeds. The mutual repelling forces of the electron’s negative charges keep them separated. The attraction of the positive charges in the nucleus balances the centrifugal force created by their motion, and stops them from simply flying out into space.
The electrons naturally fall into specific orbital patterns that vary with the number of electrons in the atom, and their relative energy levels. These orbits are usually shown as concentric layers like those shown in the picture below. Actual orbital paths are thought to be tapered ovals similar in shape to an inflated balloon.
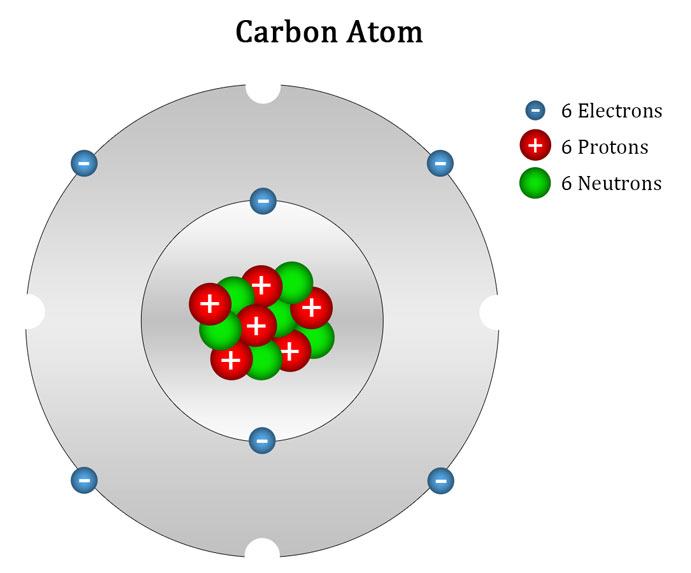
In this model of the atom, the concentric rings of orbiting electrons are called bands or shells, and all the electrons in each shell have the same energy levels. The outermost shell is called the valence shell. Only the electrons in the valence shell can interact with electrons from other atoms, so the number and behaviour of the valence electrons determines the chemical and electrical properties of the atom.
The Arrangement of the Shells
The simplest atom is hydrogen, which has only one proton and one electron. From that starting point the atoms increase in size and complexity, one proton and one electron at a time. Each atom in the progression forms one of the known elements, and those elements make up the universe.
As protons are added, the nucleus becomes heavier, and the electron orbits increase in number and complexity. These orbits develop according to specific natural laws which include the following:
- electrons can have different amounts of energy, but only in very specific increments
- electrons in each shell have a different energy level,
- the highest energy levels occur in the outer shells, farthest from the nucleus,
- electrons cannot move from shell to shell without a change in energy level,
- the inner shell fills first and cannot contain more than two electrons,
- the second shell fills next, to a maximum of eight electrons,
- the third shell can hold up to 18 electrons,
- remaining shells can have up to 32 electrons, but this maximum is affected by the number of shells above it, and
- the valance shell can never contain more than 8 electrons.
Atomic Number
All the atoms of each element contain the same number of protons in their nucleus, and that number is different for each element. Because this is a unique property, each element is assigned an atomic number that is equal to the number of protons in its nucleus. The lightest and simplest atom is hydrogen, which has only 1 proton and 1 electron, and therefore has an atomic number of 1. The atomic number for the carbon atom, shown above, is 6. Copper has an atomic number of 29, because it has 29 protons.
Free Electrons
When an atom has a full valence shell (eight electrons), it has no tendency to interact with other atoms. It is a very stable element, that is chemically inert (will not react with other elements) and will not conduct electrical current. Atoms with less than eight electrons have a strong tendency to form chemical bonds with other atoms, in an attempt to reach the stability associated with a full valence shell. In some atoms with less than four valance electrons, these outer electrons are able to take on additional energy and escape the parent atom. These free electrons do not break free from the material, but they drift through it with almost no restriction because they are able to move from one atom to another.
Free electrons are most plentiful in materials such as copper and silver that have only one valence electron, but they are also present in aluminum which has three valence electrons. Free electrons are the working fluid of our electrical systems. They allow solid materials to conduct electrical current.
Conductors
When a material contains free electrons, (or electrons that are easily freed) it is called a conductor of electricity. The best conductors have the most free electrons per unit volume of the material. For example, a cubic centimetre of copper contains approximately 8.5 x 1022 free electrons. Silver has about 5% more free electrons per unit volume, and is about 5% better as a conductor than copper.
Most metals are good conductors. Silver is the best conductor by volume, copper is a close second, but it is more widely used because of availability and cost. Aluminium is the best by weight, which is one of the reasons it is preferred for aerial conductors.
The ability of these conductors to easily pass current can be determined by four basic factors:
- Material
- Cross-sectional area
- Length
- Temperature
Material
Most metals are good conductors while most non-metallic elements are not good conductors. Silver is the best conductor. The most common conductor materials are copper and aluminum.
Cross-sectional area
A conductor with a large cross-sectional area will carry a larger current than one with a small cross-sectional area. This is why wire is available in different sizes or gauges. A high gauge number indicates a small cross-sectional area of wire. For example, 18 gauge wire is smaller than 10 gauge wire.
Length
A long conductor has a greater resistance to current flow than a short conductor. That is why there are limits to the effective length of a conductor for a given gauge.
Temperature
As the temperature of a conductor increases, the resistance will also increase, reducing conductivity.
Insulators
If it is very difficult to free electrons in a material, it will not conduct electricity so it is classed as an insulator. Atoms with five to eight valence electrons tend to be insulators. Examples include inert gases such as helium.
Semiconductors
In most conductive materials the current is carried by free electrons. Semiconductors do not contain free electrons, but the bond between the valance electrons and the nucleus is relatively weak. An electrical potential creates “free” electrons by breaking these bonds and allowing electrons to form new bonds with other atoms.
All semiconductors have 4 valance electrons. In their pure form they are neither good conductors nor good insulators. They take on more interesting properties when they are contaminated with trace amounts of other elements. Examples of semiconductors include carbon, silicon, germanium, tin, and lead. The most commonly used semiconductor is silicon. In natural form it is sand. In refined form, it is the basis of our modern electronics industry.
Static electricity and electric current
Static electricity and electric current are two separate phenomena. They both involve electric charge, and they may occur simultaneously in the same object. Static electricity refers to the electric charge of an object and the related electrostatic discharge when two objects are brought together that are not at equilibrium. An electrostatic discharge creates a change in the charge of each of the two objects. In contrast, electric current is the flow of electric charge through an object, which produces no net loss or gain of electric charge.
Electric charge
A body can possess a positive charge, a negative charge, or no charge at all. If it possesses no charge at all, it is said to be neutral. The type of charge a body has depends on the numbers of electrons compared to stable protons the body has.
Note that an electron has a mass that is a fraction of that of a proton. Also, the protons are very firmly bound to the nucleus. Therefore, it is the electrons, not the protons, that are added or removed. There are three ways in which a body can get an electric charge: by friction or contact, by conduction, and by induction.
Electric current
Free electrons are the basis of electric current. Free electrons can move between atoms. If this electron drift can be organized to move in one direction, it will produce an electron flow or electric current.
When an electron leaves an atom, it creates an electrical imbalance. If a copper atom has 29 electrons and 29 protons, it has no charge. If one electron is pulled out of the valence shell, there will be 28 electrons and 29 protons.
If there are more protons than electrons, the atom will have a net positive charge of +1 (29 protons – 28 electrons). This atom is now called a positive ion since it is no longer balanced. An ion is an atom or molecule with a positive (+) or negative (–) net electric charge, due to the loss or gain of one or more electrons.
The electron that is left attaches itself to a neighbouring copper atom and produces a net charge in that atom of –1. The positive ion will then attract another electron because it has a positive charge and opposite charges attract.
This continuous electron motion can be produced by providing an electrical push or force. The push or force that creates this flow of electrons is known as electromotive force (EMF). This force is also known by the more common name of voltage. You can measure the amount of force exerted by using an instrument called a voltmeter.
When voltage is applied to a group of atoms and electron movement begins, that movement is called induced flow or amperage. You can measure the amount of induced flow or current flow with an instrument called an ammeter. Resistance to electron movement can be measured with a test instrument called an ohmmeter.
If you line up billiard balls next to each other and strike the first one, the billiard balls transmit the motion through each other, and the ball on the end will move (Figure 4). This is how the motions of the free electrons act in a wire.
Transmission of impulse
Sources of electrical force
You have just learned that if there is a surplus of electrons at one end of a conductor and a deficiency at the other end, a current flows in the conductor. There are devices that create this difference in charge so that a current will flow. These devices are referred to as sources of electromotive force. These sources include:
- chemical
- electromagnetic induction
- friction
- heat
- pressure
- light
Chemical
A battery is a source of electrical force due to the chemical reaction that takes place between plates and an electrolyte. This reaction causes a buildup of positive ions on one plate and negative ions on the other plate. This electrical difference between the plates is also known as potential difference.
Electromagnetic induction
Electric force can be generated by using a magnetic field. This is the method by which most of the electrical energy we use is produced. An example is an alternator or generator.
Friction
Friction can cause free electrons to move from one body to another and be stored there temporarily. When you walk across a carpet, electrons are transferred to the atoms in your body and you return them to other atoms when you touch a metallic object.
Heat
If two unlike metals are placed together and heated, they will produce electrical force. An example is the thermocouple in a furnace.
Pressure
Certain crystals will produce electricity if they are squeezed under extreme pressure. An example is a barbecue starter (also called piezoelectric generator).
Light
Some crystals and semiconductors will produce electrical force when they are exposed to light. An example is the photocell in a calculator.
All six of these sources of EMF achieve the same thing. They separate charge by:
- imparting energy to the electrons
- pushing them against an electrostatic field
- causing a surplus of electrons (negative charge) at one terminal of the source and a deficiency of electrons (positive charge) at the other terminal
In a sense, the process can be likened to compressing a spring. The energy stored in the compressed spring can be used later to do useful work. The same is true of the separated charges: they store energy that can be used to do useful work.
Electrical energy always comes from some other form of energy. The source of EMF is simply the device that makes the conversion from some other form of energy to electrical energy.
This information has been adapted from B.C. Open Campus Line E Electrical Fundamentals Competency E-1, available for free at https://collection.bccampus.ca/textbooks/line-e-electrical-fundamentals-competency-e-1-describe-the-basic-principles-of-electricity-bccampus-228/